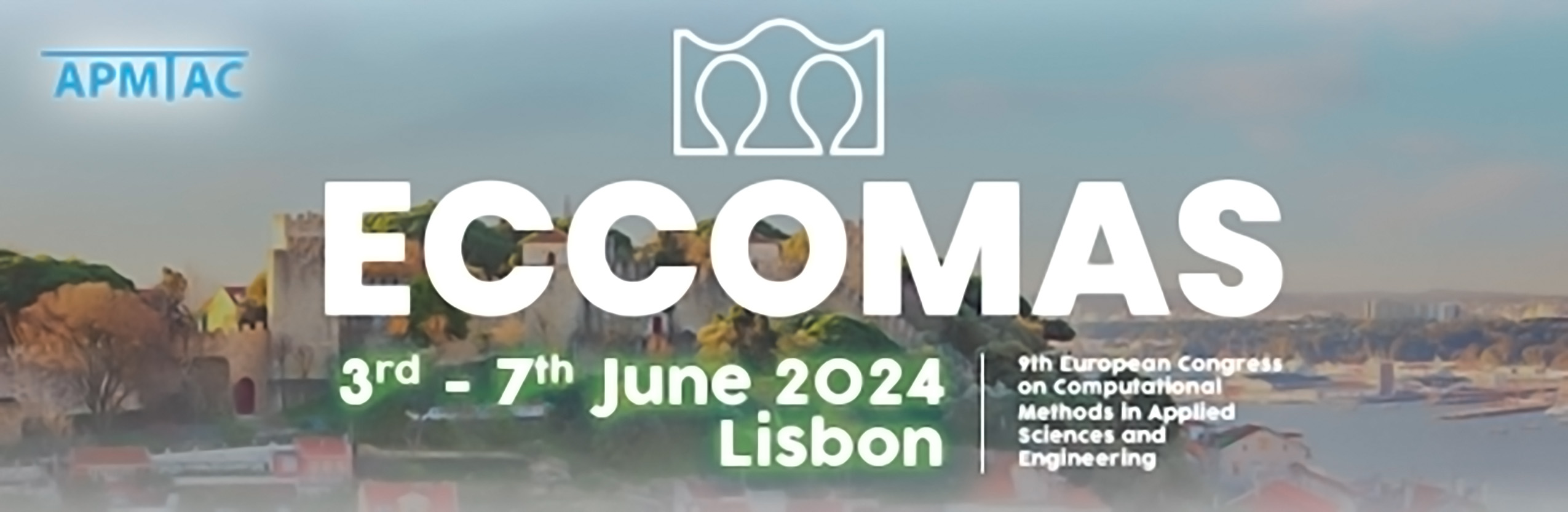
Stabilized Finite Element Methods as Physics-Compatible Discretizations for Dissipative Euler Flows
Please login to view abstract download link
Turbulent flows are expected to exhibit a kinetic energy dissipation rate that is independent of viscosity in the limit of infinite Re number. This understanding dates back to Taylor and is today known as anomalous/inviscid/inertial dissipation, or the zeroth law of turbulence. Early contributions by Kolmogorov and Onsager gave first indications that this behaviour is related to the regularity of the velocity field. Onsager conjectured that anomalous dissipation of energy may occur if the velocity field is Hölder continuous with exponent < 1/3. The phenomenon of anomalous dissipation is documented in review articles by Eyink (2008) and Dubrulle (2019). Discretization schemes for turbulent flows are often designed to be kinetic-energy-conserving in the discrete case. The one-dimensional inviscid Burgers equation with formation of shock is a simple example to understand that such schemes are, however, not suitable to describe or predict dissipative dynamics in hyperbolic transport problems. Since no energy can leave the system in the discrete (numerical) setting, energy-conserving numerical methods rule out the occurrence of inertial dissipation by construction and lead to an unphysical accumulation of energy in small scales, a phenomenon called “thermalization”. Our goal is to develop finite-element-based discretization schemes that qualify as physics-compatible in the sense that they can predict anomalous dissipation and avoid thermalization for three-dimensional incompressible Euler flows. We address this by numerical stabilization techniques and present two flavours of higher-order finite element schemes, stabilized L2-conforming methods and exactly mass-conserving H(div)-conforming methods. As compared to continuous Galerkin methods, discontinuous Galerkin methods might be seen as a natural candidate for turbulent flows since dissipation can be introduced straightforwardly by upwind-like numerical fluxes on scales near the numerical resolution limit. We showcase these developments for a classical benchmark problem, the three-dimensional inviscid Taylor-Green vortex problem at infinite Reynolds number, and interpret the results from the perspective of anomalous dissipation and singular behavior for incompressible Euler flows.