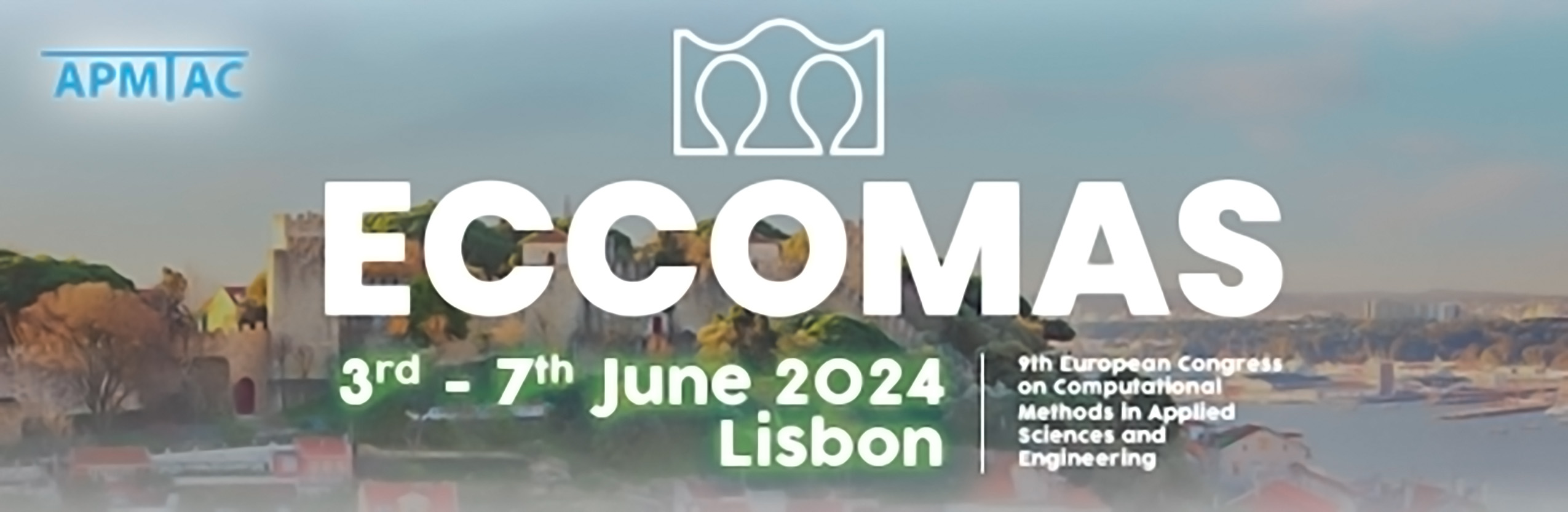
Computationally enhanced processing of 4D flow MRI for intracardiac diagnostics
Please login to view abstract download link
Monitoring intracardiac flow is essential for diagnosing and predicting cardiac-related pathologies. 4D Flow MRI offers time-resolved flow measurements over the cardiac volume, enabling the evaluation of intricate flow structures and changes in flow parameters that characterize cardiac function with reduced operator dependency. However, challenges arise as the Signal-to-Noise Ratio (SNR) declines with higher spatial resolution demands, and errors from phase wrapping, intravoxel phase dispersion, and partial volume effects due to improper segmentation of flow regions impact the accuracy of flow readouts. We developed a comprehensive processing pipeline incorporating computational fluid dynamics methodologies to address those issues and enhance the analysis of intracardiac 4D-flow MRI, tackling the following three key stages of the analysis: (1) dynamically mask velocity fields, (2) correct velocities affected by partial volume effects and noise, and (3) derive hemodynamic quantities. Masking involves creating a temporally averaged flow mask using Pseudo Complex Difference. This is refined with a time-dependent segmentation from a spatially resolved MRI Sequence. Manual registration is performed with coarse segmentation, followed by automatic adjustments using the Coherent Point Drift algorithm. Velocity correction is performed in multiple steps; first by Universal Outlier Detection to remove spurious vectors [1], and second by a global optimization scheme that enforces mass conservation [2]. To address the underprediction of velocity gradients from partial volume effects and low spatial resolution, the bias error in the velocity measurements is estimated and subtracted to obtain a more accurate field [3]. Essential hemodynamic parameters, including pressure [4] and wall shear stress [5], are computed from the masked, corrected velocity fields. To improve the hemodynamic evaluation’s robustness and counter the effects of low SNR and partial volume, these methods employ dynamic weighing schemes utilizing global minimization of uncertainty and reinforcement of flow constraints that outweigh the core flow over near-wall regions. Our approach allows for obtaining flow regions in the rapidly moving heart walls, addressing misalignments, removing spurious vectors, correcting velocities, and estimating biases to enhance accuracy for objective hemodynamic assessment.